What is Controlled Impedance?
Controlled impedance refers to the precise management of the impedance of electrical transmission lines, such as printed Circuit board (PCB) traces or cables, to match the characteristic impedance of the system. Impedance, measured in ohms (Ω), is the total opposition a circuit presents to the flow of alternating current (AC) at a given frequency. It is a complex quantity that combines resistance, capacitance, and inductance.
In a controlled impedance environment, the goal is to maintain a consistent impedance throughout the signal path. This is achieved by carefully designing the physical characteristics of the transmission lines, such as trace width, thickness, spacing, and dielectric properties of the substrate material.
Types of Controlled Impedance
There are three main types of controlled impedance:
-
Single-ended Impedance: This refers to the impedance between a single trace and its reference plane, such as a ground plane or power plane. Common single-ended impedance values include 50Ω, 75Ω, and 100Ω.
-
Differential Impedance: Differential impedance is the impedance between two traces that form a differential pair. It is important for high-speed differential signaling, such as in USB, HDMI, or Ethernet interfaces. Typical differential impedance values range from 90Ω to 120Ω.
-
Common Mode Impedance: Common mode impedance is the impedance between the two traces of a differential pair and their reference plane. It is essential for maintaining signal integrity and reducing electromagnetic interference (EMI).
The Importance of Controlled Impedance
Controlled impedance is crucial for several reasons:
Signal Integrity
In high-speed digital systems, signals propagate as electromagnetic waves along transmission lines. If the impedance of the transmission line does not match the characteristic impedance of the system, signal reflections occur at the impedance mismatch points. These reflections can lead to signal distortion, ringing, overshoot, and undershoot, which can compromise the integrity of the transmitted data.
By maintaining a consistent impedance throughout the signal path, controlled impedance minimizes signal reflections and ensures the proper transmission and reception of digital signals. This is particularly important for high-speed interfaces like DDR memory, PCI Express, and SerDes (Serializer/Deserializer) links.
Timing and Synchronization
Controlled impedance also plays a vital role in maintaining accurate timing and synchronization in digital systems. When signals encounter impedance mismatches, they experience delays and phase shifts, which can lead to timing errors and synchronization issues.
In applications like memory interfaces or high-speed parallel buses, precise timing and synchronization are essential for reliable data transfer. Controlled impedance helps to minimize signal delays and skew, ensuring that signals arrive at their destinations within the specified timing requirements.
Electromagnetic Compatibility (EMC)
Controlling impedance is also important for achieving electromagnetic compatibility (EMC) in electronic systems. EMC refers to the ability of a device to operate properly in its electromagnetic environment without causing or being susceptible to electromagnetic interference (EMI).
Impedance mismatches can cause signal reflections that lead to the radiation of electromagnetic energy, resulting in EMI. By maintaining controlled impedance, designers can minimize the generation and coupling of unwanted electromagnetic emissions, thus improving the EMC performance of the system.
Power Integrity
Controlled impedance is not limited to signal transmission lines; it also applies to power delivery networks (PDNs). In modern electronic devices, the power supply must provide stable and clean power to the various components while minimizing voltage fluctuations and noise.
The impedance of the power delivery network, including the power planes, decoupling capacitors, and interconnects, must be carefully controlled to ensure proper power distribution. Impedance mismatches in the PDN can lead to voltage droops, ground bounce, and other power integrity issues that can affect the performance and reliability of the system.
Designing for Controlled Impedance
Achieving controlled impedance requires careful design considerations and coordination between the PCB layout, material selection, and manufacturing processes.
PCB Stack-up and Material Selection
The PCB stack-up, which refers to the arrangement of conductive layers and dielectric materials, plays a crucial role in determining the impedance of the transmission lines. The thickness and Dielectric constant (Dk) of the substrate material, as well as the height and width of the traces, directly impact the impedance.
When designing for controlled impedance, the PCB stack-up must be carefully engineered to achieve the desired impedance values. This involves selecting appropriate materials with known dielectric properties and specifying the precise dimensions of the traces and spacing.
Trace Geometry and Spacing
The geometry of the traces, including their width, thickness, and spacing, is a critical factor in achieving controlled impedance. The trace width and thickness determine the cross-sectional area of the conductor, which affects its resistance and inductance. The spacing between traces and their reference planes influences the capacitance.
To achieve the target impedance, designers use impedance calculation tools and formulas to determine the optimal trace geometry based on the PCB stack-up and material properties. These calculations take into account factors such as the dielectric constant, trace width, trace thickness, and spacing.
Manufacturing Considerations
Achieving controlled impedance also requires close collaboration with the PCB Manufacturer. The manufacturing process must be tightly controlled to ensure that the actual impedance of the fabricated board matches the designed values.
PCB manufacturers use specialized equipment and techniques to maintain consistent trace geometries and dielectric properties throughout the production process. This includes precise control of etching, plating, and lamination processes to minimize variations in trace width, thickness, and spacing.
Manufacturers also employ impedance testing methods, such as time-domain reflectometry (TDR) or impedance-controlled coupons, to verify that the fabricated PCBs meet the specified impedance requirements. These tests help to ensure the quality and reliability of the final product.
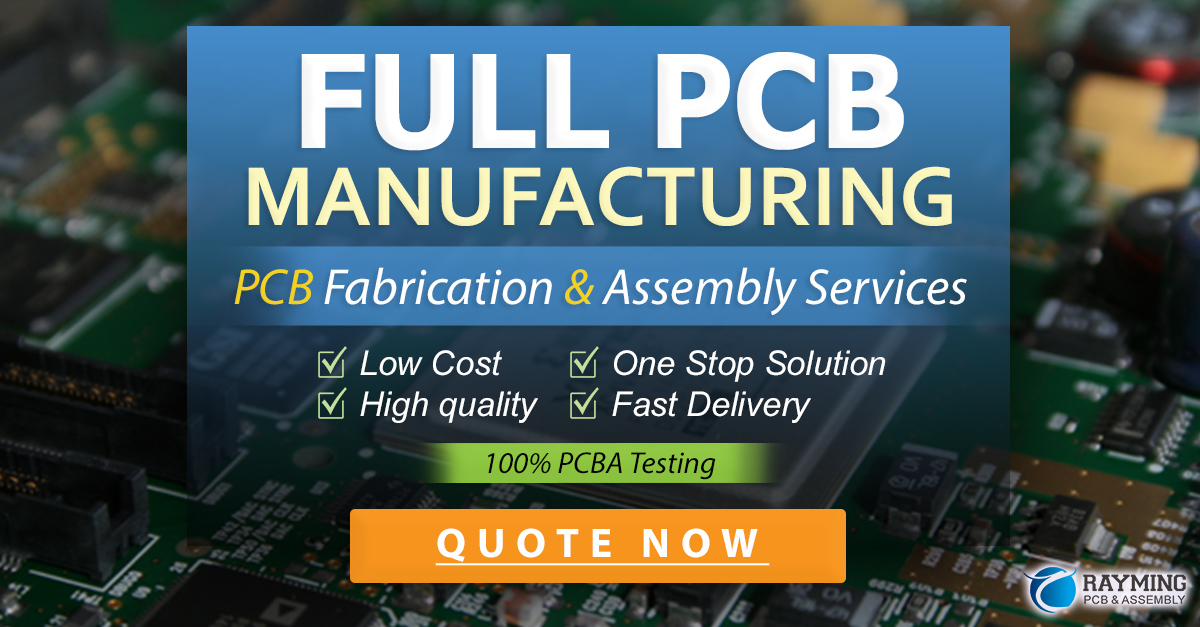
Common Applications of Controlled Impedance
Controlled impedance is essential in a wide range of electronic applications, particularly those involving high-speed digital interfaces or sensitive analog signals. Some common applications include:
High-Speed Digital Interfaces
- DDR Memory: DDR (Double Data Rate) memory interfaces, such as DDR3, DDR4, and DDR5, require controlled impedance to ensure reliable data transfer at high speeds.
- PCI Express (PCIe): PCIe is a high-speed serial interface used for interconnecting components in computers and other electronic devices. Controlled impedance is critical for maintaining signal integrity in PCIe lanes.
- USB: USB (Universal Serial Bus) interfaces, especially high-speed variants like USB 3.0 and USB 4, rely on controlled impedance for proper signal transmission.
- HDMI and DisplayPort: These digital video interfaces use controlled impedance to ensure high-quality video and audio transmission.
- Ethernet: Gigabit Ethernet and higher-speed variants require controlled impedance for reliable data communication.
RF and Wireless Applications
- Antennas: Controlled impedance is essential for designing and matching antennas to their feed lines, ensuring efficient power transfer and minimizing reflections.
- RF PCBs: In radio frequency (RF) circuits, controlled impedance is crucial for maintaining signal integrity and preventing unwanted reflections or losses.
- Wireless Modules: Controlled impedance is important for the proper functioning of wireless modules, such as Wi-Fi, Bluetooth, or cellular modems.
Analog and Mixed-Signal Circuits
- Audio Systems: In high-quality audio systems, controlled impedance is necessary for maintaining the fidelity and clarity of audio signals.
- Video Processing: Controlled impedance is important in video processing circuits to ensure the accurate transmission and synchronization of video signals.
- Sensor Interfaces: In applications involving sensitive analog sensors, controlled impedance helps to minimize signal distortion and noise.
Challenges and Considerations
Designing for controlled impedance presents several challenges and considerations that engineers must address:
Manufacturing Tolerances
Despite the best efforts of PCB manufacturers, there will always be some variations in the fabricated boards due to manufacturing tolerances. These variations can affect the actual impedance of the transmission lines, leading to deviations from the designed values.
Designers must take these tolerances into account and specify appropriate impedance ranges or tolerance bands to ensure that the manufactured boards meet the required specifications. This may involve working closely with the PCB manufacturer to understand their capabilities and limitations.
Signal Integrity Simulations
To ensure the reliable performance of high-speed digital systems, designers often rely on signal integrity simulations. These simulations help to predict the behavior of signals in the presence of impedance discontinuities, crosstalk, and other factors that can impact signal quality.
Signal integrity simulation tools, such as SPICE or electromagnetic field solvers, enable designers to analyze the effects of impedance mismatches, trace routing, and termination strategies on signal propagation. By performing these simulations, designers can optimize the PCB layout and component placement to minimize signal integrity issues.
Cost Considerations
Designing for controlled impedance can impact the overall cost of the PCB. The need for precise trace geometries, specialized materials, and tighter manufacturing tolerances can increase the fabrication and assembly costs.
Designers must balance the cost considerations with the performance requirements of the system. In some cases, it may be necessary to relax the impedance specifications or explore alternative design approaches to meet cost constraints while still maintaining acceptable signal integrity.
EMC Compliance
Achieving controlled impedance is not only important for signal integrity but also for meeting electromagnetic compatibility (EMC) regulations. Electronic devices must comply with EMC standards to ensure that they do not cause harmful interference to other devices and can operate reliably in their intended electromagnetic environment.
Designers must consider the impact of controlled impedance on EMC performance, including the potential for radiated emissions or susceptibility to external electromagnetic interference. Proper grounding, shielding, and filtering techniques may be necessary to mitigate EMC issues related to impedance mismatches.
Frequently Asked Questions (FAQ)
-
What is the difference between single-ended and differential impedance?
Single-ended impedance refers to the impedance between a single trace and its reference plane, while differential impedance is the impedance between two traces that form a differential pair. Single-ended impedance is important for signals referenced to a common ground or power plane, while differential impedance is critical for high-speed differential signaling. -
How does controlled impedance affect signal integrity?
Controlled impedance helps to minimize signal reflections caused by impedance mismatches. By maintaining a consistent impedance throughout the signal path, designers can ensure the proper transmission and reception of high-speed digital signals, reducing signal distortion, ringing, and other artifacts that can compromise signal integrity. -
What factors determine the impedance of a transmission line?
The impedance of a transmission line is determined by several factors, including the trace width, trace thickness, dielectric constant of the substrate material, and the spacing between the trace and its reference plane. These physical characteristics influence the resistance, capacitance, and inductance of the transmission line, which together determine its impedance. -
How is controlled impedance achieved in PCB manufacturing?
Controlled impedance is achieved in PCB manufacturing by carefully controlling the fabrication process to maintain consistent trace geometries and dielectric properties. This involves precise control of etching, plating, and lamination processes, as well as the use of specialized materials with known dielectric constants. PCB manufacturers also employ impedance testing methods to verify that the fabricated boards meet the specified impedance requirements. -
What are some common applications that require controlled impedance?
Controlled impedance is essential in a wide range of electronic applications, including high-speed digital interfaces (e.g., DDR memory, PCI Express, USB), RF and wireless systems (e.g., antennas, RF PCBs), and analog and mixed-signal circuits (e.g., audio systems, video processing). In general, any application that involves the transmission of high-speed signals or sensitive analog signals can benefit from controlled impedance design.
Conclusion
Controlled impedance is a fundamental concept in electronic design that plays a vital role in ensuring the integrity, reliability, and performance of modern electronic systems. By carefully managing the impedance of transmission lines, designers can minimize signal reflections, maintain accurate timing and synchronization, and achieve electromagnetic compatibility.
Achieving controlled impedance requires a combination of careful design, material selection, and manufacturing processes. Designers must consider factors such as PCB stack-up, trace geometry, and manufacturing tolerances to ensure that the actual impedance of the fabricated board matches the designed values.
As electronic systems continue to push the boundaries of speed and complexity, the importance of controlled impedance will only continue to grow. By understanding the principles and techniques involved in controlled impedance design, engineers can create robust and reliable systems that meet the ever-increasing demands of modern technology.
Impedance Type | Description | Typical Values |
---|---|---|
Single-ended Impedance | Impedance between a single trace and its reference plane | 50Ω, 75Ω, 100Ω |
Differential Impedance | Impedance between two traces forming a differential pair | 90Ω to 120Ω |
Common Mode Impedance | Impedance between the two traces of a differential pair and their reference plane | Varies based on design requirements |
No responses yet