Introduction to RF-PCB
Radio Frequency (RF) signals are electromagnetic waves that propagate through space and are used for wireless communication. In the context of Printed Circuit Boards (PCBs), RF signals are high-frequency electrical signals that are transmitted through the conductive traces on the board. RF-PCB refers to the design and fabrication of PCBs specifically optimized for handling RF signals.
Importance of RF-PCB Design
Proper RF-PCB design is crucial for ensuring the integrity and quality of RF signals. Some key reasons why RF-PCB design is important include:
-
Signal Integrity: RF signals are sensitive to noise, interference, and distortion. Proper RF-PCB design helps maintain signal integrity by minimizing signal loss, reflections, and crosstalk.
-
Impedance Matching: RF circuits require precise impedance matching to ensure maximum power transfer and minimize reflections. RF-PCB design techniques, such as controlled impedance traces and matching networks, help achieve proper impedance matching.
-
Electromagnetic Compatibility (EMC): RF-PCBs must comply with EMC regulations to prevent electromagnetic interference (EMI) with other electronic devices. Appropriate RF-PCB design practices, such as proper grounding, shielding, and filtering, help mitigate EMI issues.
-
Performance and Reliability: Well-designed RF-PCBs exhibit better performance and reliability compared to poorly designed ones. Optimal RF-PCB design ensures stable and consistent RF signal transmission, leading to improved system performance and reduced failures.
RF-PCB Design Considerations
When designing RF-PCBs, several key considerations must be taken into account to ensure optimal performance. Some of these considerations include:
1. Material Selection
The choice of PCB material is critical for RF applications. The dielectric constant (Dk) and dissipation factor (Df) of the material directly impact the signal propagation and losses. Common RF-PCB materials include:
Material | Dielectric Constant (Dk) | Dissipation Factor (Df) |
---|---|---|
FR-4 | 4.3 – 4.7 | 0.02 – 0.03 |
Rogers RO4003C | 3.38 | 0.0027 |
Rogers ro4350B | 3.48 | 0.0037 |
Isola IS680-280 | 2.80 | 0.0028 |
2. Trace Geometry
The geometry of the conductive traces on the RF-PCB plays a significant role in signal propagation and impedance matching. Some key aspects of trace geometry include:
-
Trace Width: The width of the trace determines its characteristic impedance. Proper trace width calculation is essential for achieving the desired impedance, typically 50 ohms for RF applications.
-
Trace Spacing: Adequate spacing between traces is necessary to minimize crosstalk and coupling between adjacent signals.
-
Trace Length: The length of the traces should be minimized to reduce signal loss and phase delay. Matching trace lengths is important for maintaining signal integrity and preventing timing issues.
3. Grounding and Shielding
Proper grounding and shielding techniques are crucial for RF-PCB design to minimize noise, interference, and EMI. Some important considerations include:
-
Ground Plane: A solid ground plane beneath the RF traces helps provide a low-impedance return path for the signals and shields them from external noise.
-
Via Stitching: Placing vias at regular intervals along the ground plane helps maintain a continuous ground reference and prevents ground bounce.
-
Shielding: Metallic shielding enclosures or compartments can be used to isolate sensitive RF circuits from external interference and prevent EMI.
4. Component Placement
The placement of components on the RF-PCB is critical for optimizing signal paths and minimizing parasitics. Some guidelines for component placement include:
-
Short Signal Paths: Components should be placed as close as possible to minimize the length of signal traces and reduce losses.
-
Decoupling Capacitors: Decoupling capacitors should be placed close to the power pins of active components to provide a low-impedance path for high-frequency noise.
-
Sensitive Components: Sensitive RF components, such as amplifiers and filters, should be placed away from noisy digital circuits to minimize interference.
5. Impedance Matching
Impedance matching is essential for maximizing power transfer and minimizing signal reflections in RF circuits. Some techniques for impedance matching on RF-PCBs include:
-
Controlled Impedance Traces: Designing traces with specific geometries to achieve the desired characteristic impedance, typically 50 ohms.
-
Matching Networks: Using passive components, such as capacitors and inductors, to create matching networks that transform impedances and optimize power transfer.
-
Stub Matching: Employing open or shorted stubs of specific lengths to compensate for impedance mismatches and reflections.
RF-PCB Fabrication and Assembly
The fabrication and assembly of RF-PCBs require specialized processes and techniques to ensure the desired performance and reliability. Some key aspects of RF-PCB fabrication and assembly include:
1. Multilayer PCBs
RF-PCBs often employ multilayer structures to accommodate complex RF circuits and provide shielding and grounding. Some considerations for multilayer RF-PCBs include:
-
Layer Stackup: The arrangement of signal, ground, and power layers in the PCB stackup affects signal integrity and EMC performance.
-
Blind and Buried Vias: Using blind and buried vias can help optimize signal routing and minimize the impact of via stubs on RF performance.
-
Impedance Control: Maintaining consistent impedance across layers is crucial for preventing signal reflections and ensuring proper signal propagation.
2. Controlled Impedance Manufacturing
Fabricating RF-PCBs with controlled impedance requires precise control over the manufacturing process parameters. Some key aspects of controlled impedance manufacturing include:
-
Dielectric Thickness: Maintaining consistent dielectric thickness is essential for achieving the desired characteristic impedance.
-
Copper Thickness: The thickness of the copper traces affects the impedance and current-carrying capacity of the RF traces.
-
Etching Process: Accurate etching is necessary to maintain the desired trace geometries and impedance.
3. RF-Specific Assembly Techniques
Assembling RF-PCBs requires special techniques to minimize the impact of parasitics and ensure proper RF performance. Some RF-specific assembly techniques include:
-
Hand Soldering: Hand soldering of RF components is often preferred to minimize the introduction of parasitics and ensure precise control over the soldering process.
-
Grounding and Shielding: Proper grounding and shielding techniques, such as the use of conductive gaskets and shielding cans, are employed during assembly to maintain signal integrity and prevent EMI.
-
Coaxial Connectors: RF-PCBs often incorporate coaxial connectors, such as SMA or BNC, for external RF connections. Proper connector mounting and grounding are critical for optimal RF performance.
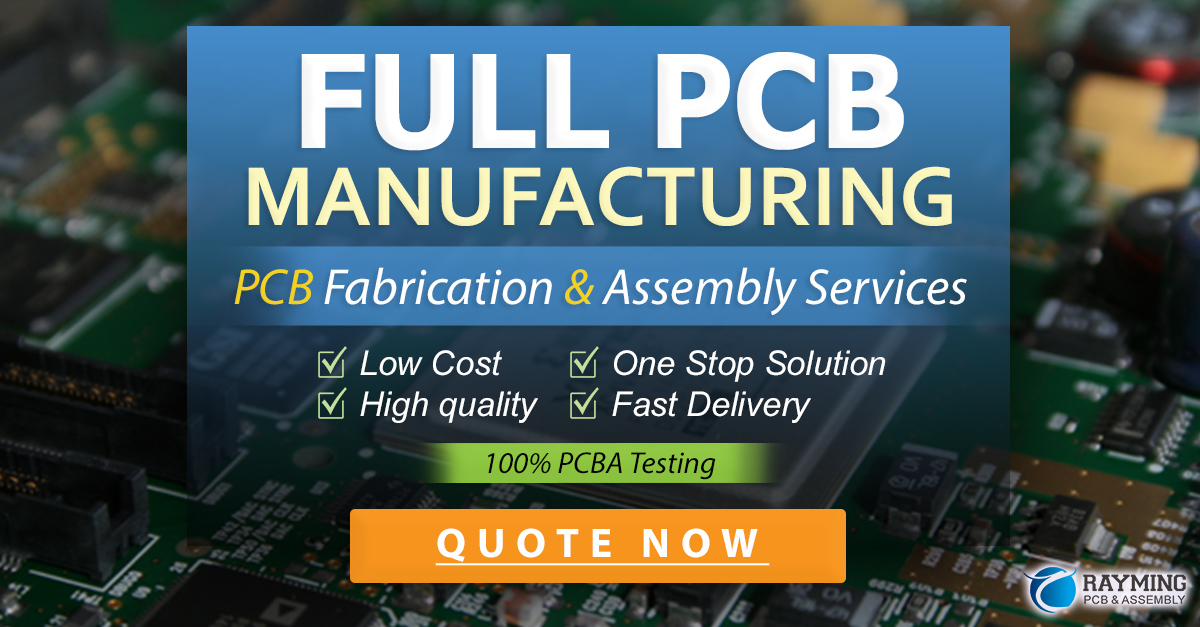
RF-PCB Testing and Validation
Testing and validation of RF-PCBs are essential to ensure that they meet the desired performance specifications and comply with relevant standards. Some common RF-PCB testing and validation techniques include:
1. Network Analyzer Measurements
A vector network analyzer (VNA) is used to measure the scattering parameters (S-parameters) of the RF-PCB. S-parameters provide information about the transmission, reflection, and impedance characteristics of the RF circuit. Some key measurements include:
-
Return Loss (S11): Measures the amount of signal reflected back from the input port, indicating impedance matching.
-
Insertion Loss (S21): Measures the signal attenuation from the input to the output port, indicating the overall signal loss.
-
Isolation (S31, S41): Measures the signal leakage between different ports, indicating the level of crosstalk and isolation.
2. Spectrum Analyzer Measurements
A spectrum analyzer is used to measure the frequency-domain characteristics of the RF-PCB. Some common spectrum analyzer measurements include:
-
Harmonic Distortion: Measures the presence of harmonics in the RF signal, indicating the level of nonlinear distortion.
-
Spurious Emissions: Measures the presence of unwanted frequency components in the RF signal, indicating the level of EMI and noise.
-
Adjacent Channel Power: Measures the power leakage into adjacent frequency channels, indicating the spectral efficiency of the RF circuit.
3. EMC Testing
EMC testing is performed to ensure that the RF-PCB complies with electromagnetic compatibility standards and regulations. Some common EMC tests include:
-
Radiated Emissions: Measures the electromagnetic emissions from the RF-PCB to ensure they are within acceptable limits.
-
Conducted Emissions: Measures the electromagnetic emissions conducted through the power and signal lines of the RF-PCB.
-
Immunity Tests: Evaluates the ability of the RF-PCB to operate correctly in the presence of external electromagnetic disturbances.
Frequently Asked Questions (FAQ)
-
What is the difference between RF-PCB and regular PCB?
RF-PCBs are specifically designed and optimized for handling high-frequency RF signals, while regular PCBs are designed for general-purpose electronic circuits. RF-PCBs employ specialized materials, trace geometries, and design techniques to ensure signal integrity and minimize losses at RF frequencies. -
What is the typical frequency range for RF-PCBs?
The frequency range for RF-PCBs can vary depending on the specific application. Generally, RF-PCBs are designed to operate from a few megahertz (MHz) to several gigahertz (GHz). Common RF frequency bands include the ISM band (2.4 GHz), Wi-Fi bands (2.4 GHz and 5 GHz), and cellular bands (800 MHz to 5 GHz). -
What are the most common materials used for RF-PCBs?
The most common materials used for RF-PCBs are: - FR-4: A glass-reinforced epoxy laminate, suitable for low-cost and general-purpose RF applications.
- Rogers RO4000 series: High-performance laminate materials with low dielectric constant and dissipation factor, ideal for high-frequency RF applications.
-
Isola materials: A range of low-loss laminate materials designed for RF and microwave applications.
-
How does the choice of dielectric material affect RF-PCB performance?
The dielectric material of the RF-PCB has a significant impact on signal propagation and losses. The dielectric constant (Dk) affects the signal velocity and wavelength, while the dissipation factor (Df) determines the amount of signal loss. Materials with lower Dk and Df are preferred for RF applications to minimize signal distortion and attenuation. -
What are some common RF-PCB design challenges?
Some common RF-PCB design challenges include: - Maintaining signal integrity and minimizing losses at high frequencies.
- Achieving proper impedance matching and minimizing reflections.
- Minimizing crosstalk and coupling between adjacent signals.
- Ensuring electromagnetic compatibility and complying with EMC regulations.
- Optimizing component placement and signal routing for optimal RF performance.
Conclusion
RF-PCB design and fabrication are critical aspects of modern wireless communication systems. Understanding the principles and techniques involved in RF-PCB design is essential for engineers and designers working on RF projects. By considering factors such as material selection, trace geometry, grounding and shielding, component placement, and impedance matching, designers can create RF-PCBs that exhibit optimal performance and reliability.
Proper fabrication and assembly techniques, along with thorough testing and validation, ensure that RF-PCBs meet the desired specifications and comply with relevant standards. As wireless technologies continue to advance, the importance of RF-PCB design will only grow, making it a crucial skill for engineers in the field of RF and microwave electronics.
By following best practices and staying up-to-date with the latest techniques and technologies, designers can create high-quality RF-PCBs that enable reliable and efficient wireless communication systems.
No responses yet