Understanding Polyimide
Polyimide is a type of polymer that belongs to the class of thermoplastics. It is synthesized by the condensation reaction of dianhydrides and diamines, resulting in a highly ordered and rigid molecular structure. The chemical structure of polyimide consists of imide groups (-CO-NR-CO-) that alternate with aromatic rings, providing the material with its unique properties.
Chemical Structure of Polyimide
The general chemical structure of polyimide can be represented as follows:
O O
|| ||
/\/\/\/\/C\/\/\/\/\/N\/\/\/\/\
|| ||
O O
The aromatic rings in the structure contribute to the thermal stability and mechanical strength of polyimide, while the imide groups provide chemical resistance and high glass transition temperature.
Mechanical Properties of Polyimide
Polyimide exhibits excellent mechanical properties, making it suitable for various demanding applications. Some of the key mechanical properties of polyimide include:
- High tensile strength
- High modulus of elasticity
- Low coefficient of thermal expansion
- Good dimensional stability
- Excellent creep resistance
However, the brittleness of polyimide is a concern in certain applications where impact resistance or flexibility is required.
Factors Affecting Polyimide brittleness
Several factors can influence the brittleness of polyimide, including:
-
Molecular Structure: The rigid and ordered molecular structure of polyimide contributes to its high strength and thermal stability but also makes it inherently brittle. The aromatic rings and imide groups in the polymer backbone restrict the mobility of the chains, resulting in a brittle behavior.
-
Glass Transition Temperature (Tg): Polyimide has a high glass transition temperature, typically ranging from 300°C to 400°C. Below the Tg, polyimide is in a glassy state, where the polymer chains have limited mobility, leading to brittle behavior. Above the Tg, polyimide becomes more flexible and less brittle.
-
Moisture Absorption: Polyimide can absorb moisture from the environment, which can plasticize the polymer and reduce its brittleness. However, excessive moisture absorption can lead to swelling and dimensional changes, affecting the mechanical properties of the material.
-
Processing Conditions: The processing conditions, such as the curing temperature and time, can impact the brittleness of polyimide. Incomplete curing or improper processing can result in residual stresses and defects, making the material more susceptible to brittle failure.
-
Additives and Fillers: The incorporation of additives and fillers can modify the mechanical properties of polyimide, including its brittleness. For example, the addition of elastomeric toughening agents can improve the impact resistance and reduce the brittleness of polyimide.
Measuring Polyimide Brittleness
The brittleness of polyimide can be evaluated through various mechanical tests, such as:
-
Tensile Test: The tensile test measures the stress-strain behavior of polyimide. A brittle material will exhibit a low elongation at break and a sharp drop in stress after reaching the yield point.
-
Impact Test: Impact tests, such as Izod or Charpy impact tests, assess the energy absorbed by polyimide during sudden loading. Brittle materials have low impact strength and absorb less energy before fracture.
-
Flexural Test: The flexural test determines the bending properties of polyimide. Brittle materials have a low flexural strain at break and may exhibit a sudden failure under bending loads.
The following table summarizes the typical values of mechanical properties for polyimide:
Property | Value Range |
---|---|
Tensile Strength (MPa) | 70-150 |
Elongation at Break (%) | 5-15 |
Flexural Modulus (GPa) | 2.5-4.0 |
Izod Impact Strength (J/m) | 20-50 |
It is important to note that these values can vary depending on the specific grade of polyimide and the testing conditions.
Strategies to Reduce Polyimide Brittleness
Several strategies can be employed to reduce the brittleness of polyimide and improve its toughness:
Toughening Agents
The incorporation of toughening agents, such as elastomers or thermoplastic particles, can enhance the impact resistance and reduce the brittleness of polyimide. These agents absorb energy during impact and promote plastic deformation, preventing brittle failure. Common toughening agents used in polyimide include:
- Rubber particles (e.g., polybutadiene, silicone rubber)
- Thermoplastic particles (e.g., polyetherimide, polysulfone)
- Core-shell particles (e.g., rubber core with a rigid shell)
The selection of the toughening agent depends on the specific requirements of the application, such as the desired mechanical properties, thermal stability, and chemical resistance.
Copolymerization
Copolymerization involves the synthesis of polyimide with different monomers to modify its molecular structure and improve its toughness. By incorporating flexible or non-aromatic monomers into the polymer backbone, the chain mobility can be increased, reducing the brittleness of the material. Examples of monomers used for copolymerization include:
- Aliphatic diamines (e.g., hexamethylenediamine)
- Ether-containing diamines (e.g., oxydianiline)
- Flexible dianhydrides (e.g., bis(3,4-dicarboxyphenyl) ether dianhydride)
Copolymerization allows for the tailoring of the mechanical properties of polyimide to meet specific application requirements.
Blending
Blending polyimide with other polymers can also improve its toughness and reduce brittleness. By mixing polyimide with a more flexible or ductile polymer, the overall mechanical properties of the blend can be enhanced. Common polymers used for blending with polyimide include:
- Polyetherimide (PEI)
- Polysulfone (PSU)
- Polyethersulfone (PES)
- Polyamide (PA)
The blending ratio and compatibility of the polymers play a crucial role in determining the final properties of the blend.
Processing Optimization
Optimizing the processing conditions can help minimize the brittleness of polyimide. Proper curing temperature and time ensure complete imidization and reduce residual stresses in the material. Controlled cooling rates after processing can also prevent the formation of internal stresses that may lead to brittle behavior.
Additionally, using appropriate molding techniques, such as compression molding or injection molding, can help achieve uniform and defect-free polyimide parts, reducing the risk of brittle failure.
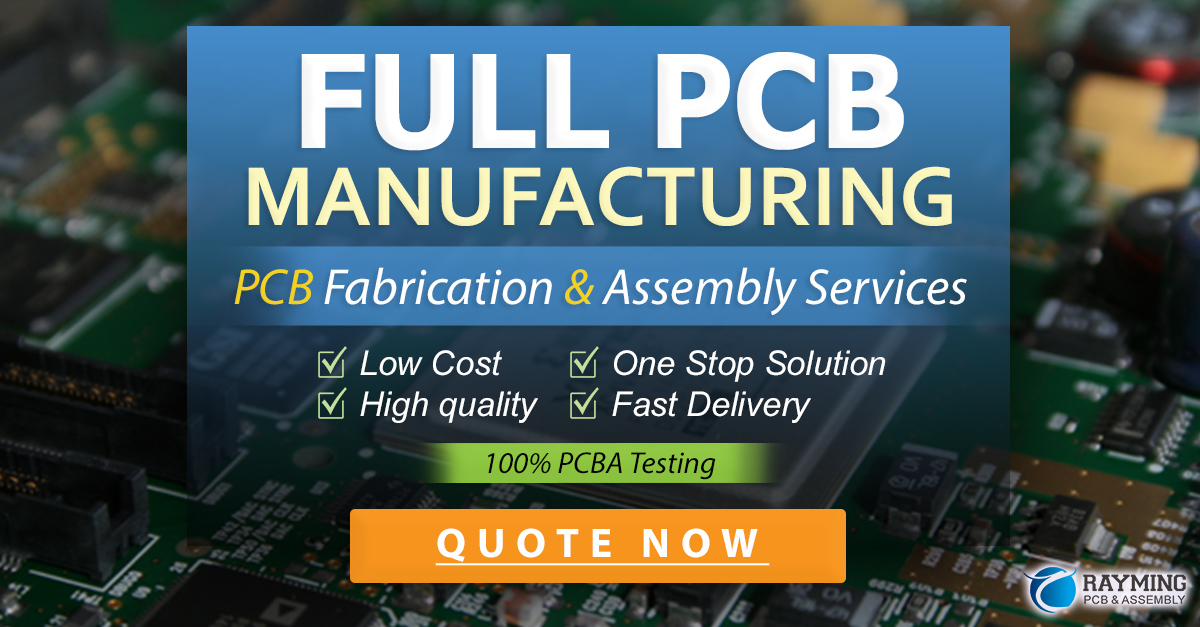
Applications of Polyimide
Despite its inherent brittleness, polyimide finds extensive use in various industries due to its exceptional thermal stability, chemical resistance, and mechanical properties. Some of the key applications of polyimide include:
Aerospace
Polyimide is widely used in the aerospace industry for high-temperature applications, such as:
- Wire and cable insulation
- Thermal insulation blankets
- Structural composites
- Adhesives and coatings
The ability of polyimide to withstand extreme temperatures and maintain its mechanical properties makes it suitable for use in spacecraft, satellites, and aircraft components.
Electronics
Polyimide is a preferred material for electronic applications due to its excellent electrical insulation properties and thermal stability. It is used in:
- Flexible printed circuit boards (PCBs)
- Chip packaging
- Display devices (e.g., OLEDs)
- Insulating films and substrates
Polyimide’s low dielectric constant, high breakdown voltage, and resistance to thermal and chemical degradation make it an ideal choice for electronic components.
Automotive
In the automotive industry, polyimide is used for high-temperature and demanding applications, such as:
- Engine components (e.g., gaskets, seals)
- Transmission parts
- Electrical insulation
- Brake pads
Polyimide’s ability to withstand high temperatures, mechanical stresses, and chemical exposure ensures reliable performance in automotive environments.
Medical
Polyimide is used in medical devices and implants due to its biocompatibility, chemical resistance, and mechanical properties. Applications include:
- Catheter tubing
- Surgical instruments
- Implantable sensors
- Prosthetic devices
Polyimide’s stability and resistance to sterilization processes make it suitable for use in medical applications.
Frequently Asked Questions (FAQ)
-
Is polyimide always brittle?
No, polyimide is not always brittle. The brittleness of polyimide depends on various factors, such as its molecular structure, glass transition temperature, moisture absorption, processing conditions, and the presence of additives or fillers. While polyimide has an inherent tendency to be brittle due to its rigid molecular structure, strategies like toughening agents, copolymerization, blending, and processing optimization can be employed to reduce its brittleness and improve its toughness. -
Can polyimide be used in applications requiring impact resistance?
Yes, polyimide can be used in applications requiring impact resistance with appropriate modifications. Toughening agents, such as elastomers or thermoplastic particles, can be incorporated into polyimide to enhance its impact resistance and reduce brittleness. Copolymerization with flexible monomers or blending with more ductile polymers can also improve the impact resistance of polyimide. However, the specific requirements of the application, such as the desired level of impact resistance and other mechanical properties, need to be considered when selecting the appropriate modification strategy. -
How does the glass transition temperature affect the brittleness of polyimide?
The glass transition temperature (Tg) of polyimide plays a significant role in its brittleness. Below the Tg, polyimide is in a glassy state, where the polymer chains have limited mobility, resulting in brittle behavior. In this state, polyimide exhibits high strength and stiffness but low elongation and impact resistance. Above the Tg, polyimide transitions to a rubbery state, where the polymer chains have increased mobility, making the material more flexible and less brittle. The Tg of polyimide typically ranges from 300°C to 400°C, depending on its specific composition and structure. -
Can processing conditions influence the brittleness of polyimide?
Yes, processing conditions can significantly influence the brittleness of polyimide. Incomplete curing or improper processing can result in residual stresses and defects in the material, making it more susceptible to brittle failure. Proper curing temperature and time are crucial to ensure complete imidization and minimize residual stresses. Controlled cooling rates after processing can also help prevent the formation of internal stresses that may lead to brittle behavior. Optimization of molding techniques, such as compression molding or injection molding, can help achieve uniform and defect-free polyimide parts, reducing the risk of brittle failure. -
What are some common applications where polyimide’s brittleness is not a major concern?
Polyimide’s brittleness is not a major concern in applications where its exceptional thermal stability, chemical resistance, and mechanical properties are of primary importance. Some common applications where polyimide’s brittleness is not a limiting factor include: -
High-temperature electrical insulation: Polyimide is widely used for wire and cable insulation in aerospace, automotive, and industrial applications due to its excellent thermal stability and electrical insulation properties.
- Electronic substrates: Polyimide films are used as substrates for flexible printed circuit boards (PCBs) and chip packaging due to their dimensional stability, low dielectric constant, and resistance to thermal and chemical degradation.
- Structural composites: Polyimide-based composites are used in aerospace and high-performance applications where high strength, stiffness, and thermal stability are required, such as in aircraft components and spacecraft structures.
- Coatings and adhesives: Polyimide coatings and adhesives are used in various industries for their excellent thermal and chemical resistance, even in environments where brittleness is not a primary concern.
In these applications, the inherent brittleness of polyimide is often manageable, and its unique combination of properties makes it a suitable choice despite its brittle nature.
Conclusion
Polyimide is a high-performance polymer known for its exceptional thermal stability, chemical resistance, and mechanical properties. While polyimide has an inherent tendency to be brittle due to its rigid molecular structure, various strategies can be employed to reduce its brittleness and improve its toughness. Toughening agents, copolymerization, blending, and processing optimization are some of the approaches used to enhance the impact resistance and flexibility of polyimide.
Despite its brittleness, polyimide finds extensive use in industries such as aerospace, electronics, automotive, and medical, where its unique combination of properties is highly valued. By understanding the factors influencing polyimide’s brittleness and employing appropriate modification strategies, engineers and material scientists can tailor the mechanical properties of polyimide to meet the specific requirements of different applications.
As research and development in polyimide chemistry continue to advance, new formulations and processing techniques are being developed to further enhance the toughness and reduce the brittleness of this remarkable polymer. With its exceptional properties and versatility, polyimide will undoubtedly continue to play a crucial role in shaping the future of high-performance materials and engineering applications.
No responses yet