Introduction to RF PCB Design
Designing a radio frequency (RF) printed circuit board (PCB) requires careful consideration of various factors to ensure optimal performance and reliability. RF PCB design involves dealing with high-frequency signals, which present unique challenges compared to traditional low-frequency digital or analog circuit design. This article will provide a comprehensive guide on how to design an RF PCB, covering key aspects such as material selection, layout techniques, signal integrity, and manufacturing considerations.
What is an RF PCB?
An RF PCB is a specialized type of printed circuit board designed to handle high-frequency signals, typically in the range of hundreds of megahertz (MHz) to several gigahertz (GHz). These circuits are commonly used in wireless communication systems, radar applications, and high-speed digital devices.
Why is RF PCB Design Important?
Proper RF PCB design is crucial for several reasons:
-
Signal Integrity: High-frequency signals are sensitive to various factors such as impedance mismatches, crosstalk, and electromagnetic interference (EMI). Careful design techniques are necessary to maintain signal integrity and minimize signal degradation.
-
Reliability: Well-designed RF PCBs ensure reliable operation of the circuit over its intended lifespan. Poor design practices can lead to signal distortion, excessive heat generation, and premature component failure.
-
Performance: Optimal RF PCB design maximizes the performance of the circuit by minimizing losses, reducing noise, and ensuring proper impedance matching. This results in better signal quality, increased range, and improved overall system performance.
RF PCB Material Selection
Substrate Materials
Choosing the right substrate material is crucial for RF PCB design. The substrate acts as the foundation for the circuit and plays a significant role in determining the electrical and mechanical properties of the board. Some common substrate materials used in RF PCB design include:
-
FR-4: FR-4 is a popular and cost-effective substrate material for general-purpose PCBs. However, it has limitations in terms of high-frequency performance due to its relatively high dielectric loss and low thermal conductivity.
-
Rogers Materials: Rogers Corporation offers a range of high-performance substrate materials specifically designed for RF applications. These materials, such as RO4000 series and RT/duroid series, offer excellent electrical properties, including low dielectric loss, stable dielectric constant, and low moisture absorption.
-
PTFE (Teflon): PTFE-based substrates, such as Taconic TLY and TLX series, provide exceptional high-frequency performance. They have very low dielectric loss, high thermal stability, and low moisture absorption. However, PTFE materials are more expensive and challenging to manufacture compared to other substrates.
Dielectric Properties
When selecting a substrate material for RF PCB design, several key dielectric properties should be considered:
-
Dielectric Constant (Dk): The dielectric constant represents the ability of a material to store electrical energy. A stable and consistent dielectric constant is important for maintaining impedance control and signal integrity.
-
Dissipation Factor (Df): The dissipation factor, also known as loss tangent, measures the amount of energy lost as heat in the substrate material. Lower dissipation factors are desirable for minimizing signal loss and maintaining high-frequency performance.
-
Thermal Conductivity: Thermal conductivity indicates the material’s ability to dissipate heat. Higher thermal conductivity helps in managing heat generated by components and preventing excessive temperature rise, which can affect circuit performance and reliability.
Copper Cladding
The choice of copper cladding is another important consideration in RF PCB design. Copper thickness and surface finish can impact signal propagation and losses. Some common copper cladding options include:
-
Standard Copper: Standard copper cladding, typically 1 oz (35 μm) or 2 oz (70 μm) thick, is suitable for most RF PCB designs. Thicker copper can be used for high-power applications or to improve heat dissipation.
-
Low-Profile Copper (LPC): LPC is a thinner copper cladding, typically 0.5 oz (18 μm) or less, which offers better high-frequency performance due to reduced conductor losses. However, LPC may have limitations in terms of current-carrying capacity and mechanical stability.
-
Reverse-Treated Copper (RTC): RTC is a specialized copper treatment that provides a smoother surface finish, reducing skin effect losses at high frequencies. It is commonly used in high-frequency RF PCB designs.
RF PCB Layout Techniques
Grounding and Power Distribution
Proper grounding and power distribution are essential for maintaining signal integrity and preventing unwanted coupling in RF PCB designs. Some key considerations include:
-
Ground Planes: Use solid ground planes to provide a low-impedance return path for high-frequency signals. Avoid splitting ground planes or creating discontinuities, as they can lead to signal integrity issues.
-
Power Planes: Dedicate separate power planes for different voltage levels to minimize noise coupling between power supplies. Use decoupling capacitors close to active components to provide a stable and clean power supply.
-
Via Placement: Minimize the use of vias in RF signal paths, as they can introduce discontinuities and reflections. When vias are necessary, use properly sized and spaced vias to maintain signal integrity.
Impedance Matching
Impedance matching is crucial in RF PCB design to ensure maximum power transfer and minimize signal reflections. Some techniques for impedance matching include:
-
Controlled Impedance Traces: Design traces with specific widths and spacings to achieve the desired characteristic impedance, typically 50 ohms for RF circuits. Use impedance calculators or simulation tools to determine the appropriate trace dimensions.
-
Terminations: Use proper termination techniques, such as resistive termination or matching networks, to match the impedance of the source and load to the characteristic impedance of the transmission line.
-
Stub Matching: Employ stub matching techniques, such as open or short stubs, to compensate for impedance mismatches and improve signal integrity.
Trace Routing and Spacing
Careful trace routing and spacing are essential to minimize crosstalk, coupling, and signal distortion in RF PCB designs. Some guidelines include:
-
Trace Width: Choose appropriate trace widths based on the desired characteristic impedance and current-carrying requirements. Wider traces have lower impedance and can handle higher currents but may occupy more board space.
-
Trace Spacing: Maintain adequate spacing between adjacent traces to minimize crosstalk and coupling. The spacing should be based on the signal frequency, trace geometry, and substrate properties.
-
Trace Length Matching: Match the lengths of critical signal traces to ensure equal propagation delays and maintain signal integrity. Use meandering or serpentine routing techniques to adjust trace lengths when necessary.
Component Placement
Proper component placement is crucial for optimizing signal paths, minimizing parasitics, and improving overall circuit performance. Consider the following:
-
Critical Component Placement: Place critical RF components, such as amplifiers, mixers, and filters, as close as possible to their associated circuitry to minimize trace lengths and parasitic effects.
-
Orientation and Symmetry: Orient components to minimize coupling and maintain symmetry in differential signal paths. Consider the component’s package and pinout when determining the optimal placement.
-
Shielding and Isolation: Use shielding techniques, such as metal cans or compartmentalization, to isolate sensitive RF components from potential sources of interference. Maintain proper isolation between different circuit sections to prevent unwanted coupling.
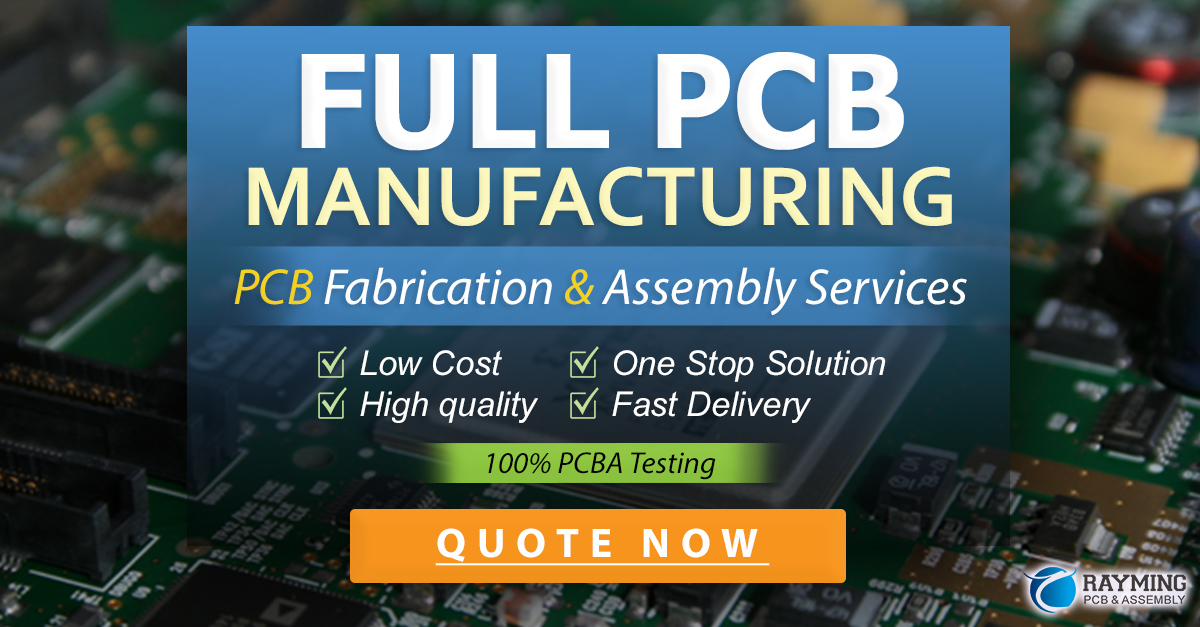
Signal Integrity Considerations
Transmission Line Effects
At high frequencies, PCB traces behave as transmission lines rather than simple conductors. Understanding and managing transmission line effects is crucial for maintaining signal integrity in RF PCB designs. Some key considerations include:
-
Characteristic Impedance: Ensure that the characteristic impedance of the transmission line matches the impedance of the source and load to prevent reflections and standing waves.
-
Propagation Delay: Consider the propagation delay of signals along the transmission line, which is determined by the trace length and the velocity of propagation in the substrate material.
-
Losses: Account for losses in the transmission line, including conductor losses (skin effect and proximity effect) and dielectric losses. Minimize losses by using appropriate trace geometries and low-loss substrate materials.
Electromagnetic Interference (EMI)
EMI can significantly impact the performance and reliability of RF PCBs. Proper EMI management techniques should be employed to minimize interference and ensure compliance with regulatory standards. Some strategies include:
-
Shielding: Use shielding techniques, such as metal enclosures or conductive coatings, to contain EMI and prevent it from affecting nearby circuits or devices.
-
Grounding and Filtering: Implement proper grounding and filtering techniques to reduce EMI. Use ground planes, bypass capacitors, and EMI filters to suppress unwanted high-frequency noise.
-
Layout Considerations: Minimize loop areas and use proper trace routing techniques to reduce the radiation and pickup of EMI. Avoid running sensitive traces parallel to high-speed or noisy traces.
Crosstalk and Coupling
Crosstalk and coupling occur when signals from one trace or component interfere with signals on adjacent traces or components. To mitigate these effects, consider the following:
-
Trace Spacing: Maintain adequate spacing between adjacent traces to minimize crosstalk. Use simulation tools to determine the appropriate spacing based on signal frequencies and trace geometries.
-
Guard Traces: Implement guard traces or ground shields between sensitive traces to reduce coupling. Guard traces help to isolate signals and prevent unwanted interactions.
-
Differential Signaling: Use differential signaling techniques for critical RF signals to reduce the impact of common-mode noise and crosstalk. Differential traces should be closely spaced and have equal lengths to maintain signal integrity.
Manufacturing Considerations
PCB Fabrication
When designing an RF PCB, it is important to consider the capabilities and limitations of the PCB fabrication process. Some key aspects to consider include:
-
Minimum Feature Size: Ensure that the design adheres to the minimum feature sizes supported by the PCB manufacturer, such as minimum trace width, spacing, and via size.
-
Layer Stack-up: Work closely with the PCB manufacturer to define an appropriate layer stack-up that meets the requirements of the RF design, including the number of layers, dielectric thicknesses, and copper weights.
-
Tolerances: Understand the manufacturing tolerances for critical dimensions, such as trace widths and spacings, and design the PCB accordingly. Tight tolerances may increase manufacturing costs.
Assembly and Soldering
Proper assembly and soldering techniques are crucial for ensuring the reliability and performance of RF PCBs. Consider the following:
-
Component Placement: Use machine-plaable components whenever possible to ensure accurate and consistent placement. Provide clear markings and orientation indicators on the PCB to facilitate correct component placement.
-
Soldering: Select appropriate soldering techniques based on the component packages and PCB design. Use reflow soldering for surface-mount components and wave soldering or hand soldering for through-hole components. Follow recommended soldering profiles and guidelines.
-
Inspection and Testing: Implement thorough inspection and testing procedures to verify the quality of the assembled PCB. Perform visual inspections, automated optical inspections (AOI), and electrical tests to identify any defects or performance issues.
Testing and Validation
Comprehensive testing and validation are essential to ensure that the RF PCB meets its intended performance and reliability requirements. Some key testing considerations include:
-
Functional Testing: Perform functional tests to verify that the RF PCB operates as expected, including tests for signal integrity, power consumption, and overall system performance.
-
Environmental Testing: Conduct environmental tests to assess the PCB’s ability to withstand various operating conditions, such as temperature, humidity, and vibration. These tests help to ensure the reliability and durability of the design.
-
EMC Testing: Perform electromagnetic compatibility (EMC) tests to ensure that the RF PCB complies with relevant EMC standards and regulations. This includes tests for radiated emissions, conducted emissions, and susceptibility to external electromagnetic interference.
Frequently Asked Questions (FAQ)
1. What is the difference between an RF PCB and a regular PCB?
An RF PCB is specifically designed to handle high-frequency signals, typically in the range of hundreds of megahertz (MHz) to several gigahertz (GHz). It requires special design considerations, such as material selection, impedance matching, and signal integrity management, to ensure optimal performance. Regular PCBs, on the other hand, are designed for lower-frequency digital or analog circuits and may not have the same stringent requirements as RF PCBs.
2. What are the most common substrate materials used for RF PCBs?
Some of the most common substrate materials used for RF PCBs include:
– FR-4: A cost-effective and widely used substrate material, suitable for general-purpose PCBs but with limitations at high frequencies.
– Rogers Materials: High-performance substrate materials specifically designed for RF applications, offering low dielectric loss and stable dielectric constant.
– PTFE (Teflon): PTFE-based substrates provide exceptional high-frequency performance, low dielectric loss, and high thermal stability, but are more expensive and challenging to manufacture.
3. Why is impedance matching important in RF PCB design?
Impedance matching is crucial in RF PCB design to ensure maximum power transfer and minimize signal reflections. When the impedance of the source, transmission line, and load are properly matched, signal integrity is maintained, and unwanted reflections and standing waves are minimized. Impedance mismatches can lead to signal distortion, reduced power transfer efficiency, and potential damage to RF components.
4. What are some techniques for reducing EMI in RF PCB designs?
Some techniques for reducing EMI in RF PCB designs include:
– Shielding: Using metal enclosures or conductive coatings to contain EMI and prevent it from affecting nearby circuits or devices.
– Grounding and Filtering: Implementing proper grounding and filtering techniques, such as ground planes, bypass capacitors, and EMI filters, to suppress unwanted high-frequency noise.
– Layout Considerations: Minimizing loop areas, using proper trace routing techniques, and avoiding running sensitive traces parallel to high-speed or noisy traces to reduce the radiation and pickup of EMI.
5. What are the key considerations for manufacturing RF PCBs?
When manufacturing RF PCBs, some key considerations include:
– PCB Fabrication: Ensuring that the design adheres to the minimum feature sizes supported by the PCB manufacturer, defining an appropriate layer stack-up, and understanding manufacturing tolerances for critical dimensions.
– Assembly and Soldering: Using machine-placeable components, selecting appropriate soldering techniques based on component packages and PCB design, and following recommended soldering profiles and guidelines.
– Testing and Validation: Performing comprehensive functional testing, environmental testing, and EMC testing to ensure that the RF PCB meets its intended performance and reliability requirements.
Conclusion
Designing an RF PCB requires careful consideration of various factors, including material selection, layout techniques, signal integrity, and manufacturing considerations. By understanding the unique challenges associated with high-frequency circuits and following best practices in RF PCB design, engineers can create robust and reliable circuits that meet the demanding requirements of modern wireless and high-speed applications.
Effective RF PCB design involves selecting appropriate substrate materials with suitable dielectric properties, implementing proper grounding and power distribution techniques, and employing impedance matching and trace routing strategies to maintain signal integrity. Additionally, designers must consider the impact of EMI, crosstalk, and coupling and take appropriate measures to mitigate these effects.
Collaboration with PCB fabrication and assembly partners is essential to ensure that the design is manufacturable and meets the required specifications. Thorough testing and validation, including functional testing, environmental testing, and EMC testing, are crucial for verifying the performance and reliability of the RF PCB.
By following the guidelines and best practices outlined in this article, engineers can confidently approach the design of RF PCBs and create high-performance circuits that meet the ever-increasing demands of modern technology.
No responses yet