Introduction to PCB Inductance
Printed Circuit Boards (PCBs) are essential components in modern electronics, allowing for the interconnection of various components in a compact and efficient manner. One of the key parameters to consider when designing a PCB is the inductance of the traces. PCB inductance can have a significant impact on the performance of high-speed circuits, leading to signal integrity issues, electromagnetic interference (EMI), and power loss. In this article, we will explore the concept of PCB inductance and provide a comprehensive guide on how to calculate the inductance of PCB traces.
What is PCB Inductance?
PCB inductance is the property of a PCB trace that opposes changes in the current flowing through it. When a current flows through a PCB trace, it creates a magnetic field around the trace. This magnetic field stores energy, which is released back into the circuit when the current changes. The inductance of a PCB trace is a measure of its ability to store this magnetic energy.
Factors Affecting PCB Inductance
Several factors influence the inductance of a PCB trace, including:
- Trace length
- Trace width
- Trace thickness
- Dielectric constant of the PCB material
- Proximity to other traces or components
- Frequency of the signal
Understanding these factors is crucial for accurately calculating the inductance of PCB traces and designing high-performance circuits.
Calculating PCB Inductance
There are several methods for calculating the inductance of PCB traces, ranging from simple approximations to more complex numerical simulations. In this section, we will discuss three common methods: the microstrip inductance formula, the stripline inductance formula, and numerical simulation using electromagnetic field solvers.
Microstrip Inductance Formula
A microstrip is a type of PCB trace that consists of a conducting strip separated from a ground plane by a dielectric layer. The inductance of a microstrip trace can be approximated using the following formula:
L = 0.2 × l × [ln(2l / (w + t)) + 0.5 + 0.2235 × (w + t) / l] (nH)
Where:
– L is the inductance in nanohenries (nH)
– l is the length of the trace in inches
– w is the width of the trace in inches
– t is the thickness of the trace in inches
This formula provides a quick and easy way to estimate the inductance of a microstrip trace, but it has some limitations. It assumes that the trace is isolated from other traces and components, and it does not account for the frequency-dependent effects of the Dielectric Material.
Stripline Inductance Formula
A stripline is another type of PCB trace that consists of a conducting strip sandwiched between two ground planes, with a dielectric material separating them. The inductance of a stripline trace can be approximated using the following formula:
L = 0.2 × l × [ln(4h / (0.67 × (w + t))) + 0.5] (nH)
Where:
– L is the inductance in nanohenries (nH)
– l is the length of the trace in inches
– w is the width of the trace in inches
– t is the thickness of the trace in inches
– h is the distance between the trace and the ground planes in inches
This formula assumes that the stripline trace is isolated from other traces and components and that the dielectric material is homogeneous and lossless.
Numerical Simulation using Electromagnetic Field Solvers
For more accurate and comprehensive calculations of PCB inductance, designers often rely on numerical simulations using electromagnetic field solvers. These software tools use finite element analysis (FEA) or method of moments (MoM) techniques to solve Maxwell’s equations and calculate the electromagnetic fields around the PCB traces.
Some popular electromagnetic field solvers include:
- Ansys HFSS
- Keysight ADS
- Cadence Sigrity
- CST Studio Suite
- Altair FEKO
Using these tools, designers can create detailed 3D models of their PCB layouts and simulate the electromagnetic behavior of the traces under various conditions. This allows for the accurate calculation of PCB inductance, as well as other important parameters such as impedance, crosstalk, and EMI.
Best Practices for Minimizing PCB Inductance
While calculating PCB inductance is essential for designing high-performance circuits, it is equally important to minimize inductance wherever possible. Here are some best practices for reducing PCB inductance:
-
Keep traces short: The shorter the trace, the lower its inductance. Try to place components as close together as possible and use direct routing paths.
-
Use wider traces: Increasing the width of a trace reduces its inductance. However, this comes at the cost of increased capacitance and may not always be feasible due to space constraints.
-
Use thicker traces: Increasing the thickness of a trace also reduces its inductance. This is especially important for power and ground traces, which carry large currents.
-
Use ground planes: Placing a ground plane beneath a trace reduces its inductance by providing a low-impedance return path for the current. This also helps to reduce EMI and improve signal integrity.
-
Avoid long parallel traces: When two traces run parallel to each other for a long distance, they can form a coupled inductor, increasing the overall inductance of the circuit. Try to avoid long parallel runs or use techniques like serpentine routing to minimize coupling.
-
Use low-inductance components: When selecting components for your PCB, choose those with low inductance packages, such as surface-mount devices (SMDs) and chip-scale packages (CSPs).
By following these best practices, designers can minimize the inductance of their PCB traces and improve the overall performance of their circuits.
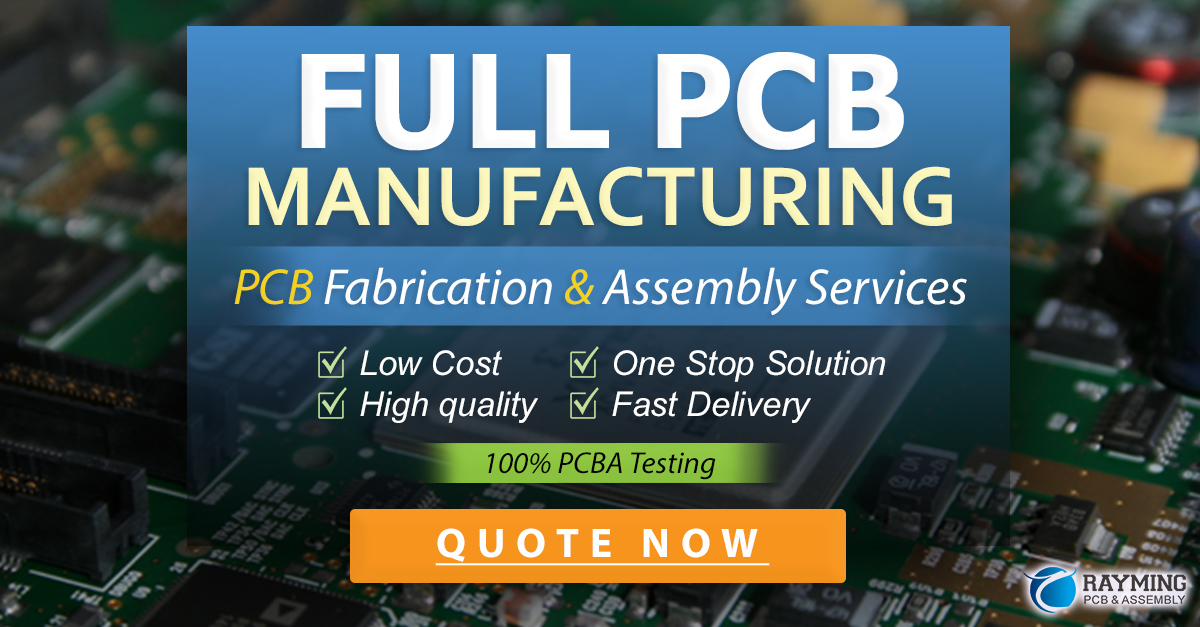
Conclusion
Calculating the inductance of PCB traces is a critical step in designing high-speed, high-performance electronic circuits. By understanding the factors that influence PCB inductance and using the appropriate calculation methods, designers can ensure that their circuits meet the desired specifications and avoid issues like signal integrity problems and EMI.
Whether using simple approximations like the microstrip and stripline inductance formulas or more advanced numerical simulations with electromagnetic field solvers, it is essential to carefully consider the inductance of PCB traces throughout the design process. Additionally, by following best practices for minimizing inductance, such as keeping traces short, using wider and thicker traces, and employing ground planes, designers can further optimize their PCB layouts for maximum performance.
As electronic devices continue to push the boundaries of speed and complexity, the accurate calculation and management of PCB inductance will only become more critical. By staying up-to-date with the latest tools, techniques, and best practices, PCB designers can ensure that their circuits are ready to meet the challenges of the future.
Frequently Asked Questions (FAQ)
1. What is the difference between microstrip and stripline PCB traces?
A microstrip trace consists of a conducting strip separated from a ground plane by a dielectric layer, while a stripline trace is a conducting strip sandwiched between two ground planes with a dielectric material separating them. Microstrip traces are easier to manufacture and offer lower capacitance, but stripline traces provide better signal integrity and EMI performance.
2. How does increasing the width of a PCB trace affect its inductance?
Increasing the width of a PCB trace reduces its inductance. This is because a wider trace has a larger cross-sectional area, which allows for a greater flow of current and a corresponding reduction in the magnetic field around the trace. However, increasing the trace width also increases its capacitance, so designers must strike a balance between inductance and capacitance when selecting trace widths.
3. What is the purpose of using a ground plane in a PCB?
A ground plane serves several purposes in a PCB. First, it provides a low-impedance return path for the current flowing through the traces, which helps to reduce the inductance of the traces. Second, it acts as a shield against electromagnetic interference (EMI), preventing unwanted signals from coupling into the traces. Finally, it helps to distribute heat evenly across the PCB, improving the thermal performance of the circuit.
4. Why is it important to consider the frequency of the signal when calculating PCB inductance?
The frequency of the signal is important when calculating PCB inductance because the inductance of a trace is frequency-dependent. At high frequencies, the skin effect causes the current to flow primarily on the surface of the trace, effectively reducing its cross-sectional area and increasing its inductance. Additionally, the dielectric material surrounding the trace may exhibit frequency-dependent properties, affecting the overall inductance of the trace. To accurately calculate PCB inductance, designers must consider the Frequency Range over which the circuit will operate.
5. What are some common issues that can arise from excessive PCB inductance?
Excessive PCB inductance can lead to several issues in electronic circuits, including:
-
Signal integrity problems: High inductance can cause reflections, ringing, and other distortions in the signal waveform, leading to data errors and reduced performance.
-
Electromagnetic interference (EMI): Inductance can cause unwanted coupling between traces, leading to crosstalk and EMI. This can interfere with the operation of nearby circuits and cause compliance issues with electromagnetic compatibility (EMC) regulations.
-
Power loss: High inductance in power distribution networks can cause voltage drops and power losses, reducing the efficiency of the circuit and potentially leading to thermal issues.
-
Timing errors: In high-speed digital circuits, excessive inductance can cause propagation delays and timing errors, leading to data corruption and system failures.
To avoid these issues, designers must carefully calculate and manage the inductance of their PCB traces, using a combination of analytical formulas, numerical simulations, and best design practices.
No responses yet