Understanding High Current Requirements
Before embarking on a high current PCB Design, it is crucial to understand the specific requirements of your application. High current PCBs are typically used in power electronics, automotive systems, industrial controls, and other applications where substantial amounts of current need to flow through the circuit board.
Defining High Current
The definition of “high current” can vary depending on the context and the specific application. However, as a general guideline, PCBs handling currents above 1 ampere (A) are often considered high current boards. The actual current levels can range from a few amperes to hundreds of amperes, depending on the system requirements.
Challenges in High Current PCB Design
Designing PCBs for high current applications presents several challenges that must be addressed to ensure proper functioning and longevity of the board. Some of the key challenges include:
- Excessive heat generation due to high power dissipation
- Voltage drops across traces and components
- Electromigration and Copper thickness limitations
- Electromagnetic interference (EMI) and signal integrity issues
By carefully considering these challenges and applying appropriate design techniques, engineers can create robust and reliable high current PCBs.
Trace Width Calculations
One of the most critical aspects of high current PCB design is determining the appropriate trace widths to handle the required current levels. Insufficient trace widths can lead to excessive heat generation, voltage drops, and potential failure of the PCB.
Factors Affecting Trace Width
Several factors influence the required trace width for a given current level:
- Current amplitude: The higher the current, the wider the trace needs to be.
- Ambient temperature: Higher ambient temperatures reduce the current-carrying capacity of traces.
- Trace thickness: Thicker traces can handle higher currents.
- Copper weight: Higher copper weights allow for higher current-carrying capacity.
- Trace length: Longer traces require wider widths to minimize voltage drops.
IPC-2152 Standard for Trace Width Calculation
The IPC-2152 standard provides guidelines for determining the appropriate trace widths based on the current level, ambient temperature, and copper thickness. This standard takes into account the temperature rise of the trace due to the current flow and ensures that the temperature remains within acceptable limits.
Here’s an example table showing the minimum trace widths for various current levels at an ambient temperature of 25°C and 1 oz. copper thickness, based on the IPC-2152 standard:
Current (A) | Minimum Trace Width (mm) |
---|---|
1 | 0.2 |
2 | 0.4 |
3 | 0.6 |
4 | 0.8 |
5 | 1.0 |
It’s important to note that these are minimum values, and it’s often recommended to use wider traces to provide an additional safety margin and improve heat dissipation.
Online Trace Width Calculators
To simplify the trace width calculation process, several online tools and calculators are available. These tools take into account various parameters such as current level, ambient temperature, copper thickness, and trace length to determine the appropriate trace width. Some popular online trace width calculators include:
- Saturn PCB Toolkit: https://saturnpcb.com/pcb_toolkit/
- EEWeb PCB Trace Width Calculator: https://www.eeweb.com/tools/external-pcb-trace-width
- CircuitCalc Trace Width Calculator: http://circuitcalc.com/wordpress/2006/01/31/pcb-trace-width-calculator/
While these calculators provide a good starting point, it’s always recommended to verify the results with the IPC-2152 standard and consult with experienced PCB designers for critical applications.
Thermal Management Techniques
Effective thermal management is crucial in high current PCB design to prevent overheating, component damage, and premature failure. Several techniques can be employed to dissipate heat and maintain acceptable operating temperatures.
Copper Pour and Plane
One of the most common techniques for thermal management is the use of copper pour or plane. By filling unused areas of the PCB with copper, you can create a large surface area for heat dissipation. The copper pour acts as a heat sink, spreading the heat generated by the high current traces and components across the board.
When using copper pour, it’s important to ensure proper connectivity to the ground plane or power plane, depending on the design requirements. Thermal vias can be used to provide a low-resistance path for heat transfer between layers.
Thermal Vias
Thermal vias are small plated holes drilled through the PCB to transfer heat from one layer to another. They are commonly used to connect high current traces or components to the copper pour or plane on the opposite side of the board. By providing a direct path for heat dissipation, thermal vias help in maintaining acceptable operating temperatures.
When placing thermal vias, it’s important to consider the following factors:
- Via size: Larger vias provide better heat transfer but occupy more board space.
- Via spacing: Adequate spacing between vias ensures proper heat dissipation without compromising signal integrity.
- Via placement: Thermal vias should be placed close to the heat-generating components or traces for optimal heat transfer.
External Heat Sinks
In some cases, the heat generated by high current components may exceed the capabilities of copper pour and thermal vias alone. In such situations, external heat sinks can be used to provide additional cooling. Heat sinks are metal structures with a large surface area that are attached to the heat-generating components to facilitate heat dissipation.
When selecting a heat sink, consider factors such as:
- Material: Aluminum and copper are common heat sink materials due to their high Thermal conductivity.
- Size and shape: The heat sink should be appropriately sized to fit the component and provide sufficient surface area for heat dissipation.
- Thermal interface material: A thermal interface material, such as thermal paste or thermal pads, is used to ensure efficient heat transfer between the component and the heat sink.
Other Thermal Management Techniques
In addition to the techniques mentioned above, other methods can be employed for thermal management in high current PCBs:
- Airflow and ventilation: Ensuring adequate airflow around the PCB can help in dissipating heat. Ventilation holes or fans can be used to improve air circulation.
- Component placement: Strategic placement of high current components can help in heat dissipation. Placing components with similar thermal characteristics together and providing sufficient spacing between them can improve overall thermal performance.
- PCB-Material/”>PCB material selection: Choosing PCB Materials with higher thermal conductivity, such as aluminum-backed PCBs or metal core PCBs, can enhance heat dissipation.
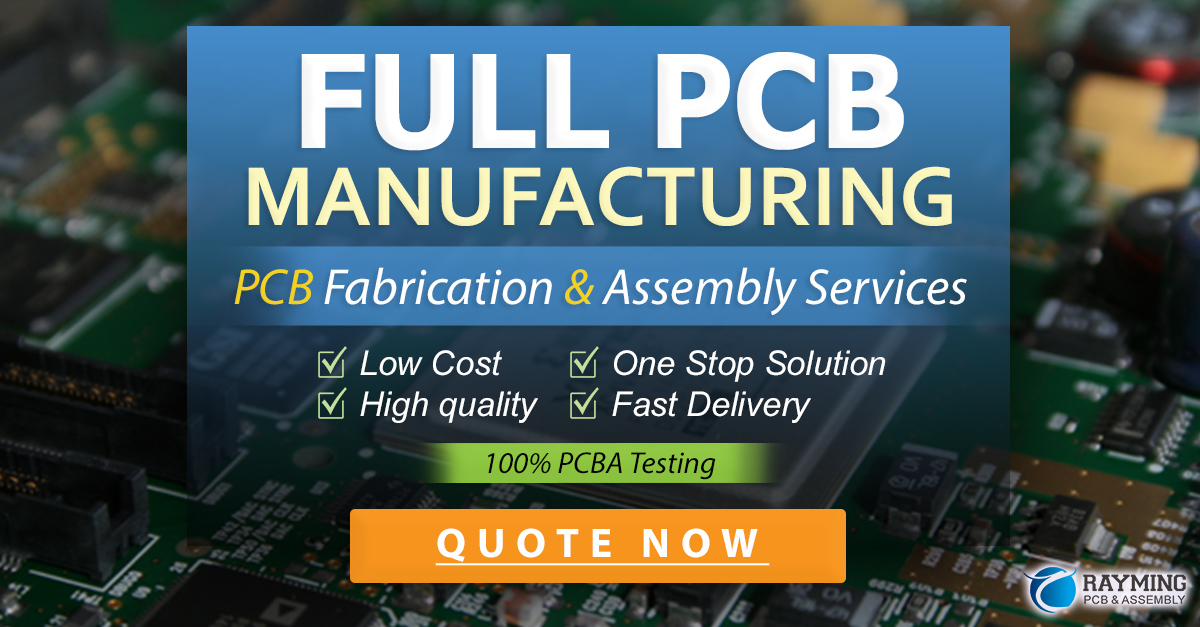
Component Selection for High Current PCBs
Proper component selection is crucial for the success of high current PCB designs. Components used in high current applications must be able to handle the required current levels and withstand the associated thermal stress.
Power Connectors
Power connectors are critical components in high current PCBs as they provide the interface for power input and output. When selecting power connectors, consider the following factors:
- Current rating: The connector should be rated for the maximum expected current level with an appropriate safety margin.
- Contact resistance: Low contact resistance ensures minimal voltage drop and heat generation at the connector.
- Mechanical strength: The connector should be able to withstand the mechanical stress associated with high current connections.
Some common power connector types used in high current applications include:
- Anderson PowerPole connectors
- XT60 connectors
- High-current terminal blocks
Fuses and Circuit Breakers
Fuses and circuit breakers are essential safety components in high current PCBs. They protect the circuit from overcurrent conditions and prevent damage to components and traces.
When selecting fuses or circuit breakers, consider the following factors:
- Current rating: The fuse or circuit breaker should be rated slightly above the maximum expected current level to provide protection without nuisance tripping.
- Voltage rating: The voltage rating should be appropriate for the system voltage.
- Response time: Fast-acting fuses or circuit breakers are preferred for high current applications to quickly interrupt fault currents.
Capacitors
Capacitors play a crucial role in high current PCBs by providing local energy storage and filtering. When selecting capacitors, consider the following factors:
- Capacitance: The capacitance value should be sufficient to provide the required energy storage and filtering.
- Voltage rating: The voltage rating should be higher than the maximum expected voltage in the circuit.
- Ripple current rating: The capacitor should be able to handle the expected ripple current without overheating.
Aluminum electrolytic capacitors and ceramic capacitors are commonly used in high current applications.
MOSFETs and IGBTs
MOSFETs (Metal-Oxide-Semiconductor Field-Effect Transistors) and IGBTs (Insulated-Gate Bipolar Transistors) are widely used in high current switching applications. When selecting these components, consider the following factors:
- Current rating: The component should be rated for the maximum expected current level with an appropriate safety margin.
- Voltage rating: The voltage rating should be higher than the maximum expected voltage in the circuit.
- Thermal characteristics: The component should have good thermal performance and be able to dissipate heat effectively.
- Switching speed: The switching speed should be appropriate for the application requirements.
Inductors and Transformers
Inductors and transformers are used in power conversion and filtering applications. When selecting these components for high current PCBs, consider the following factors:
- Current rating: The inductor or transformer should be rated for the maximum expected current level with an appropriate safety margin.
- Core material: The core material should be suitable for the frequency range and provide low losses.
- Winding wire gauge: The winding wire should be thick enough to handle the required current without excessive heating.
High Current PCB Layout Techniques
Proper PCB layout is essential for the optimal performance and reliability of high current designs. Here are some key layout techniques to consider:
Power and Ground Planes
Using dedicated power and ground planes in high current PCBs helps in reducing voltage drops, improving heat dissipation, and minimizing electromagnetic interference (EMI). By providing a low-impedance path for current flow, power and ground planes ensure stable voltage distribution across the board.
When designing power and ground planes, consider the following guidelines:
- Use thick copper layers: Thicker copper layers provide lower resistance and better current-carrying capacity.
- Minimize impedance: Keep the power and ground planes as close to each other as possible to minimize impedance and reduce noise.
- Avoid split planes: Split planes can create discontinuities and increase impedance, leading to voltage drops and EMI issues.
Component Placement
Strategic component placement is crucial for optimizing current flow and minimizing voltage drops in high current PCBs. Follow these guidelines for effective component placement:
- Place high current components close to power input: Minimize the distance between high current components and the power input to reduce trace lengths and voltage drops.
- Group components with similar current requirements: Place components with similar current requirements close together to minimize trace lengths and improve current sharing.
- Provide adequate spacing: Ensure sufficient spacing between components to facilitate heat dissipation and avoid thermal coupling.
Trace Routing
Proper trace routing is essential for minimizing voltage drops, reducing EMI, and ensuring signal integrity in high current PCBs. Consider the following guidelines:
- Use wide traces: As discussed earlier, use appropriate trace widths based on the current requirements to minimize voltage drops and ensure reliable operation.
- Minimize trace lengths: Keep high current traces as short as possible to reduce resistance and voltage drops.
- Avoid sharp bends: Sharp bends in traces can cause current crowding and increase resistance. Use gentle curves or 45-degree angles for trace routing.
- Use multiple vias: When routing high current traces between layers, use multiple vias to provide a low-resistance path and improve current-carrying capacity.
Grounding and Shielding
Proper grounding and shielding techniques are crucial for minimizing EMI and ensuring signal integrity in high current PCBs. Consider the following guidelines:
- Use a single-point ground: Implement a single-point ground strategy to avoid ground loops and minimize EMI.
- Provide sufficient grounding: Ensure adequate grounding of components and shields to minimize noise and improve signal quality.
- Use ground planes: As mentioned earlier, use ground planes to provide a low-impedance return path for currents and minimize EMI.
- Implement shielding: Use shielding techniques, such as grounded copper pours or metal enclosures, to contain EMI and protect sensitive circuits.
FAQ
Q1: What is considered a high current in PCB design?
A1: In PCB design, currents above 1 ampere (A) are typically considered high current. However, the actual current levels can vary depending on the specific application, ranging from a few amperes to hundreds of amperes.
Q2: How do I calculate the appropriate trace width for a given current level?
A2: The IPC-2152 standard provides guidelines for determining the appropriate trace widths based on factors such as current level, ambient temperature, and copper thickness. You can also use online trace width calculators or consult with experienced PCB designers for guidance.
Q3: What are some effective thermal management techniques for high current PCBs?
A3: Effective thermal management techniques for high current PCBs include using copper pour or planes, implementing thermal vias, utilizing external heat sinks, ensuring proper airflow and ventilation, strategic component placement, and selecting PCB materials with higher thermal conductivity.
Q4: What should I consider when selecting components for high current PCBs?
A4: When selecting components for high current PCBs, consider factors such as current rating, voltage rating, thermal characteristics, and mechanical strength. Choose components that can handle the required current levels and withstand the associated thermal stress.
Q5: What are some key layout techniques for high current PCBs?
A5: Key layout techniques for high current PCBs include using dedicated power and ground planes, strategic component placement, proper trace routing (using wide traces, minimizing trace lengths, avoiding sharp bends), and implementing effective grounding and shielding techniques.
By following the guidelines and techniques discussed in this article, engineers can design reliable and efficient high current PCBs that meet the specific requirements of their applications. Remember to always adhere to industry standards, use appropriate tools and calculators, and consult with experienced professionals when designing high current PCBs for critical applications.
No responses yet