Introduction to PCB
Printed Circuit Boards (PCBs) are essential components in modern electronic devices. They serve as the foundation for interconnecting electronic components and enabling the functionality of various devices, from smartphones and computers to industrial equipment and medical instruments. In this comprehensive article, we will explore the primary purposes of PCBs, their manufacturing process, types, and the key factors that contribute to their widespread use in the electronics industry.
The Primary Purposes of PCB
1. Interconnecting Electronic Components
The primary purpose of a PCB is to provide a platform for connecting electronic components. PCBs consist of a non-conductive substrate, typically made of fiberglass or other composite materials, with conductive copper traces etched onto the surface. These traces act as pathways for electrical signals to travel between components, such as resistors, capacitors, integrated circuits (ICs), and other electronic devices. By offering a reliable and organized means of interconnection, PCBs enable the efficient transfer of electrical signals and power within an electronic device.
2. Miniaturization and Space Optimization
Another crucial purpose of PCBs is to facilitate the miniaturization of electronic devices. As technology advances, the demand for smaller, more compact devices continues to grow. PCBs allow for the dense packaging of electronic components, minimizing the overall size of the device. By utilizing multi-layer PCBs, where multiple layers of conductive traces are stacked on top of each other, designers can further increase the component density and optimize the use of available space. This miniaturization not only makes devices more portable and convenient but also reduces manufacturing costs and improves overall system performance.
3. Ensuring Mechanical Stability
PCBs not only provide electrical connectivity but also offer mechanical support to the electronic components. The non-conductive substrate of the PCB acts as a stable platform on which components can be securely mounted. Through the use of through-hole technology (THT) or surface-mount technology (SMT), components are soldered onto the PCB, ensuring a strong mechanical bond. This mechanical stability is crucial for maintaining the integrity of the electronic device, especially in applications that are subject to vibrations, shocks, or environmental stresses. PCBs help prevent components from becoming loose or disconnected, thereby enhancing the reliability and longevity of the device.
4. Facilitating Mass Production
PCBs play a vital role in enabling the mass production of electronic devices. The standardization and automation of PCB manufacturing processes allow for the efficient and cost-effective production of large quantities of identical boards. PCB design software, such as computer-aided design (CAD) tools, enables designers to create precise and repeatable layouts that can be easily transferred to manufacturing. Automated assembly lines, equipped with pick-and-place machines and reflow ovens, can rapidly populate PCBs with components, significantly reducing assembly time and labor costs. This mass production capability is essential for meeting the growing demand for electronic devices in various industries.
5. Enabling Customization and Flexibility
PCBs offer a high degree of customization and flexibility in electronic design. Designers can create PCBs tailored to specific applications, incorporating unique features, form factors, and functionalities. The ability to route conductive traces in complex patterns allows for the optimization of signal integrity, power distribution, and electromagnetic compatibility (EMC). Additionally, PCBs can be designed with multiple layers, each serving a specific purpose, such as power planes, ground planes, or signal layers. This layering technique enables the separation of different types of signals, minimizing interference and improving overall system performance.
PCB Manufacturing Process
The manufacturing process of PCBs involves several key steps that ensure the quality and reliability of the final product. Let’s take a closer look at each stage:
-
PCB Design: The process begins with the creation of a PCB layout using specialized design software. Engineers and designers determine the placement of components, routing of traces, and the overall layout of the board based on the specific requirements of the electronic device.
-
Substrate Preparation: The chosen substrate material, typically a fiberglass-reinforced epoxy laminate, is cut to the desired size and shape. Copper foil is then laminated onto the substrate using heat and pressure, forming the conductive layer.
-
Drilling: Holes are drilled through the substrate at precise locations to accommodate through-hole components and provide interconnections between layers in multi-layer PCBs. Automated drilling machines ensure accuracy and repeatability.
-
Patterning: The copper layer is patterned using a photolithographic process. A photoresist layer is applied to the copper, and the desired circuit pattern is transferred onto the photoresist using UV light exposure through a photomask. The exposed areas of the photoresist are then removed, leaving the desired copper traces.
-
Etching: The exposed copper areas are etched away using a chemical solution, typically an acidic copper etchant. This process removes the unwanted copper, leaving only the desired conductive traces on the substrate.
-
Plating: If required, additional copper plating can be applied to the traces and holes to increase their thickness and improve conductivity. This step is particularly important for high-current applications or to meet specific manufacturing requirements.
-
Solder Mask Application: A solder mask, usually a green or other colored polymer, is applied to the PCB surface. This mask covers the copper traces, leaving only the exposed pads and areas where components will be soldered. The solder mask provides insulation, protection, and improves the aesthetics of the PCB.
-
Silkscreen Printing: Text, logos, and component identifiers are printed onto the PCB using a silkscreen process. This information helps in the assembly process and provides a reference for users and technicians.
-
Surface Finish: A surface finish, such as Hot Air Solder Leveling (HASL), Immersion Silver, or Electroless Nickel Immersion Gold (ENIG), is applied to the exposed copper pads. This finish protects the copper from oxidation and enhances the solderability of the pads.
-
Electrical Testing: The manufactured PCB undergoes rigorous electrical testing to ensure its functionality and reliability. Automated test equipment verifies the continuity of the traces, checks for short circuits, and confirms the proper operation of the board.
By following these manufacturing steps, PCB manufacturers can produce high-quality boards that meet the specific requirements of various electronic devices and applications.
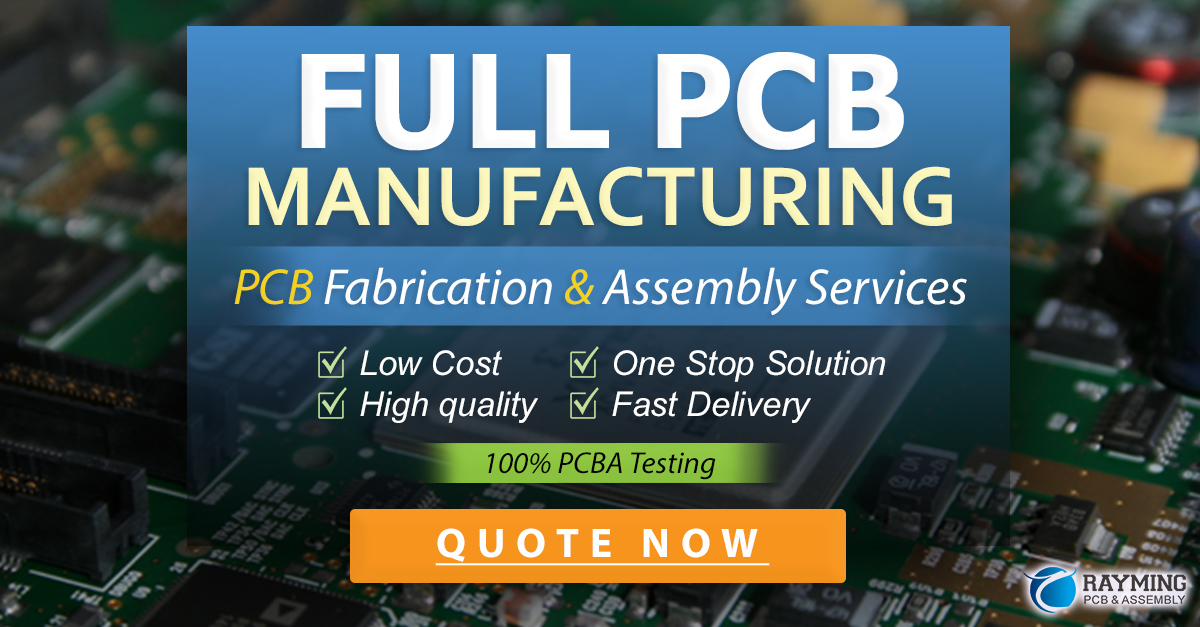
Types of PCBs
PCBs come in different types, each with its own characteristics and advantages. Let’s explore the most common types of PCBs:
-
Single-Layer PCBs: These are the simplest and most cost-effective type of PCBs. They consist of a single conductive layer, usually copper, on one side of the substrate. Single-layer PCBs are suitable for simple, low-density designs and are commonly used in basic electronic projects or prototypes.
-
Double-Layer PCBs: Double-layer PCBs have conductive layers on both sides of the substrate. The two layers are interconnected through plated-through holes (PTHs) or vias. Double-layer PCBs offer increased routing flexibility and higher component density compared to single-layer boards. They are widely used in a variety of electronic devices and applications.
-
Multi-Layer PCBs: Multi-layer PCBs consist of three or more conductive layers sandwiched between multiple layers of insulating substrate. The layers are interconnected through PTHs or vias. Multi-layer PCBs provide even greater routing flexibility and allow for the integration of complex circuitry in a compact space. They are commonly used in advanced electronic systems, such as smartphones, computers, and aerospace applications.
-
Flexible PCBs: Flexible PCBs, also known as flex circuits, are made from flexible substrate materials, such as polyimide or polyester. They can bend, twist, and conform to various shapes, making them ideal for applications that require flexibility or where space is limited. Flexible PCBs are commonly used in wearable devices, medical equipment, and aerospace systems.
-
Rigid-Flex PCBs: Rigid-flex PCBs combine the benefits of both rigid and flexible PCBs. They consist of rigid PCB sections connected by flexible PCB sections. This combination allows for three-dimensional packaging and enables the integration of multiple PCBs into a single assembly. Rigid-flex PCBs are often used in applications that require high reliability, such as aerospace, military, and medical devices.
-
High-Frequency PCBs: High-frequency PCBs are designed to handle high-speed signals and minimize signal integrity issues. They use specialized substrate materials with low dielectric constants and controlled impedance traces to minimize signal reflections and maintain signal integrity. High-frequency PCBs are essential in applications such as telecommunications, radar systems, and high-speed digital devices.
-
Metal Core PCBs: Metal core PCBs (MCPCBs) have a metal substrate, typically aluminum, instead of the traditional fiberglass substrate. The metal core provides excellent thermal conductivity, allowing for efficient heat dissipation. MCPCBs are commonly used in high-power LED lighting applications and power electronics, where heat management is crucial.
Type of PCB | Layers | Flexibility | Common Applications |
---|---|---|---|
Single-Layer PCB | 1 | Rigid | Simple electronics, prototypes |
Double-Layer PCB | 2 | Rigid | General-purpose electronics |
Multi-Layer PCB | 3 or more | Rigid | Complex devices, smartphones, computers |
Flexible PCB | Varies | Flexible | Wearables, medical devices, aerospace |
Rigid-Flex PCB | Varies | Both | High-reliability applications, 3D packaging |
High-Frequency PCB | Varies | Rigid | Telecommunications, radar, high-speed digital devices |
Metal Core PCB | Varies | Rigid | High-power LEDs, power electronics |
Key Factors Contributing to PCB Usage
Several key factors contribute to the widespread use of PCBs in the electronics industry. Let’s explore these factors in detail:
-
Reliability: PCBs offer a high level of reliability compared to other interconnection methods, such as point-to-point wiring or wire-wrap. The robust construction and precise manufacturing processes ensure stable and consistent electrical connections. PCBs can withstand vibrations, shocks, and environmental stresses, making them suitable for a wide range of applications, including industrial, automotive, and aerospace systems.
-
Cost-Effectiveness: PCBs provide a cost-effective solution for mass production of electronic devices. The standardization of PCB manufacturing processes and the use of automated assembly lines significantly reduce production costs. Additionally, the ability to integrate multiple components onto a single board minimizes the overall component count and simplifies the assembly process, further reducing costs. As a result, PCBs enable the production of affordable electronic devices for consumers.
-
Scalability: PCBs offer excellent scalability, allowing designers to create boards of various sizes and complexities. From small, single-layer boards for simple projects to large, multi-layer boards for complex systems, PCBs can be tailored to meet the specific requirements of different applications. This scalability enables the development of a wide range of electronic devices, from compact wearables to large industrial control systems.
-
Signal Integrity: PCBs are designed to maintain signal integrity, ensuring that electrical signals travel efficiently and reliably between components. Proper PCB layout techniques, such as controlled impedance traces, ground planes, and power planes, help minimize signal reflections, crosstalk, and electromagnetic interference (EMI). By maintaining signal integrity, PCBs enable the reliable operation of high-speed digital devices and sensitive analog circuits.
-
Thermal Management: PCBs play a crucial role in thermal management, especially in high-power applications. The use of metal core PCBs or the incorporation of thermal vias and heat sinks helps dissipate heat generated by electronic components. Effective thermal management prevents overheating, improves system reliability, and extends the lifespan of electronic devices.
-
Reproducibility: PCBs offer excellent reproducibility, ensuring consistent performance across multiple production runs. The standardized manufacturing processes and the use of computer-aided design (CAD) tools enable the creation of precise and repeatable PCB layouts. This reproducibility is essential for maintaining the quality and reliability of electronic devices, especially in mass production scenarios.
-
Testability: PCBs are designed with testability in mind, allowing for easy verification of functionality and troubleshooting. Test points, bed-of-nails fixtures, and boundary-scan techniques enable automated testing of PCBs during manufacturing and assembly. This testability helps identify and rectify any defects or issues early in the production process, reducing costs and improving overall product quality.
-
Regulatory Compliance: PCBs are designed to meet various regulatory requirements, such as electromagnetic compatibility (EMC), safety standards, and environmental regulations. Proper PCB design practices, such as grounding, shielding, and the use of compliant materials, help ensure that electronic devices meet the necessary regulatory standards. This compliance is crucial for the commercialization and global distribution of electronic products.
-
Continuous Advancement: The PCB industry continuously evolves and adopts new technologies and materials to meet the ever-changing demands of the electronics industry. Advancements in PCB manufacturing processes, such as high-density interconnect (HDI) technology, embedded components, and 3D printing, enable the creation of more compact, efficient, and high-performance electronic devices. These advancements drive innovation and open up new possibilities for electronic design.
Frequently Asked Questions (FAQ)
-
What is the difference between a PCB and a printed wiring board (PWB)?
A PCB and a PWB are essentially the same things. The term “printed wiring board” was used in the early days of the electronics industry, but “printed circuit board” has become the more common and widely accepted term. -
Can PCBs be recycled?
Yes, PCBs can be recycled. The recycling process involves separating the valuable materials, such as copper, gold, and other metals, from the non-metallic components. Specialized recycling facilities use various techniques, including mechanical shredding, chemical processing, and thermal treatment, to recover these materials for reuse. -
What is the typical lifespan of a PCB?
The lifespan of a PCB depends on several factors, including the quality of the materials used, the manufacturing process, the operating environment, and the specific application. In general, a well-designed and manufactured PCB can last for many years, often exceeding the lifespan of the electronic device it is used in. However, factors such as exposure to extreme temperatures, humidity, vibrations, or corrosive substances can reduce the lifespan of a PCB. -
How do I choose the right type of PCB for my project?
Choosing the right type of PCB depends on the specific requirements of your project. Consider factors such as the complexity of the circuit, the required component density, the operating environment, and the budget. Single-layer or double-layer PCBs are suitable for simple projects, while multi-layer PCBs are ideal for more complex designs. Flexible or rigid-flex PCBs are chosen when flexibility or 3D packaging is required. Consult with a PCB design expert or manufacturer to determine the most appropriate type of PCB for your project. -
What are the common challenges faced during PCB design and manufacturing?
Some common challenges in PCB design and manufacturing include: - Ensuring proper signal integrity and minimizing electromagnetic interference (EMI)
- Achieving optimal component placement and routing for high-density designs
- Managing thermal dissipation and preventing overheating
- Maintaining manufacturing tolerances and ensuring reproducibility
- Complying with regulatory requirements and industry standards
- Balancing cost, performance, and reliability considerations
Addressing these challenges requires careful design practices, collaboration with experienced PCB manufacturers, and adherence to best practices and guidelines.
Conclusion
In conclusion, PCBs play a vital role in the modern electronics industry, serving as the backbone for interconnecting electronic components and enabling the functionality of a wide range of devices. The primary purposes of PCBs include providing a reliable means of interconnection, facilitating miniaturization and space optimization, ensuring mechanical stability, enabling mass production, and offering customization and flexibility in electronic design.
The PCB manufacturing process involves several key steps, from design and substrate preparation to drilling, patterning, etching, and surface finishing. Different types of PCBs, such as single-layer, double-layer, multi-layer, flexible, rigid-flex, high-frequency, and metal core PCBs, cater to various application requirements and design challenges.
The widespread use of PCBs in the electronics industry is attributed to several key factors, including reliability, cost-effectiveness, scalability, signal integrity, thermal management, reproducibility, testability, regulatory compliance, and continuous advancement.
As technology continues to evolve, the PCB industry remains at the forefront, constantly innovating and adapting to meet the ever-changing demands of the electronics market. From consumer gadgets to industrial automation, medical devices to aerospace systems, PCBs will continue to play a crucial role in shaping the future of electronics, enabling the development of more advanced, efficient, and reliable devices.
No responses yet